Problem
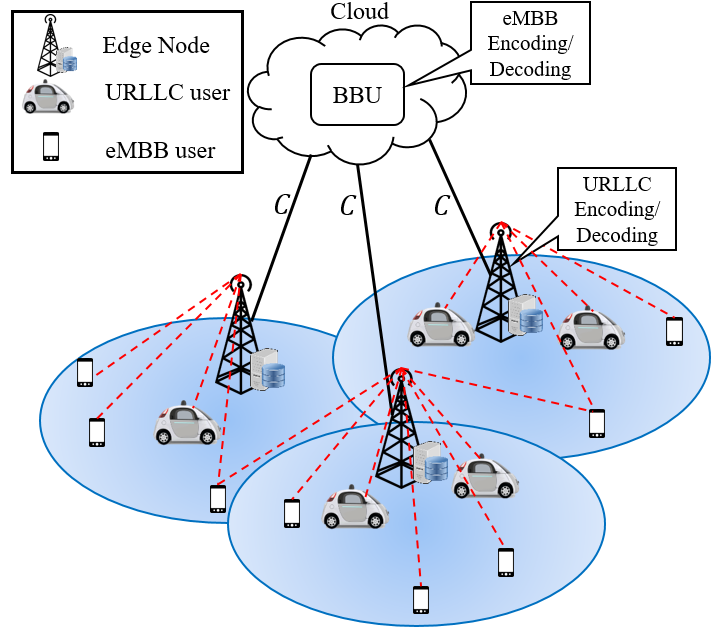
In 5G, Ultra-Reliable Low-Latency Communications (URLLC) – catering to use cases such as vehicular-to-cellular communications and Industry 4.0 — and enhanced Mobile Broadband (eMBB) – with its support of applications such as virtual reality – will share the same radio interface and network architecture. The 5G network architecture will be fog-like (see Fig. 1), enabling a flexible split of network functionalities between cloud and edge nodes. The cloud generally enables centralised processing, but at the cost of an increased latency for fronthaul transfer, while the edge can provide low-latency feedback but subject to the constraints of local processing.
This raises the following questions:
- How should radio resources be shared between the two services?
- How should the URLLC and eMBB network slices be configured?
A Novel Solution
In a recent work just published on IEEE Access , we proposed a novel solution illustrated in Fig. 1, whereby
- Baseband processing is carried out at the edge for the URLLC slice, hence ensuring low latency, and centrally at the Base Band Unit (BBU) as in a C-RAN for the eMBB slice, with the aim of increasing spectral efficiency;
- eMBB and URLLC services can share the same radio resources in a non-orthogonal fashion – an approach we define as Heterogeneous Non-Orthogonal Multiple Access.
Towards the goal of managing the interference between URLLC and eMBB packets arising from H-NOMA, we consider a number of practical approaches in order of complexity. For the uplink, we have:
- Treating URLLC interference as noise: each edge node forwards both eMBB and URLLC signal to the BBU, where the eMBB signal is decoded while treating URLLC signal as noise;
- Puncturing: each edge node discards the received eMBB signal whenever a URLLC user is transmitting;
- Successive Interference Cancellation (SIC): each edge node decodes and cancels the URLLC signal before transmitting only the eMBB signal to the cloud.
And for the downlink we consider:
- Superposition coding: each edge node transmits a superposition of both eMBB and URLLC signal to corresponding users;
- Puncturing: each edge node discards the eMBB signal whenever a URLLC signal is generated at the edge node.
It is noted that there is no counterpart of successive interference cancellation for the downlink.
Some Results
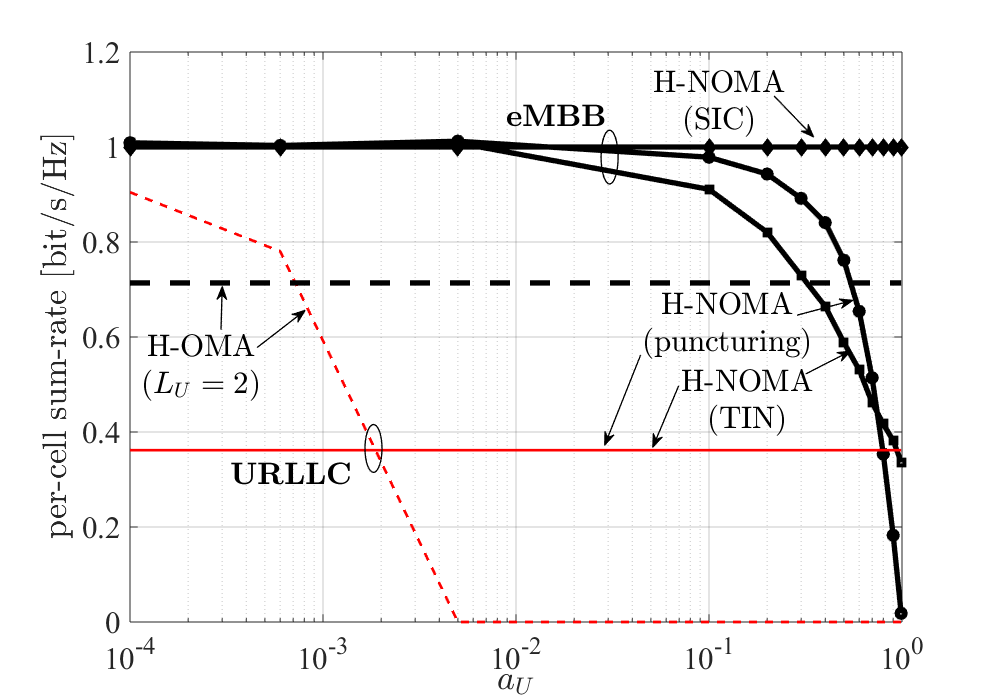
To give a taste of the results in the paper, we now provide an example. In Fig. 2, we plot the eMBB average per-cell sum-rates (black curves) and URLLC per-cell outage capacity (red curves) for the uplink as function of the URLLC activation probability. The latter is a measure of the URLLC traffic load. In general, the results demonstrate the potential advantages of H-NOMA for both services, especially when the URLLC traffic load is sufficiently large and successive interference cancellation is enabled at the edge nodes.
Link to our paper: https://ieeexplore.ieee.org/stamp/stamp.jsparnumber=8612914
Leave a Reply